The Science Behind Altitude Sickness, Hypoxia, and AMS
By Lloyd C | Updated April 23rd, 2010
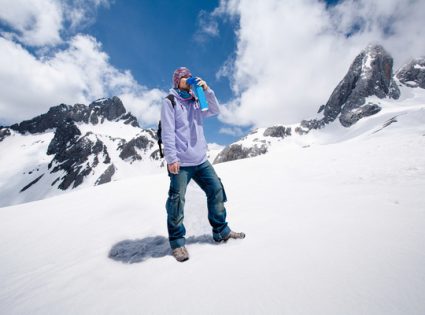
Unfortunately, there is no insurance for adventure travel, and there is always a what-if involved for personal risk.
There’s risk of injury, fatigue, exposure, and disease. But when it comes to altitude, things change.
Not only do we become more susceptible to these risks, but we expose ourselves to an additional risk: Acute Mountain Sickness (AMS).
AMS is quite literally debilitating. In addition to the typical effects of altitude (shortness of breath, sore muscles, and suppressed appetite), one can sufferer experiencing splitting headaches, rib-fracturing coughs, and a nauseous delirium. While those symptoms sound awful, there’s something even worse about AMS. Here we explore the science behind altitude sickness, hypoxia, and AMS.
An Invitation to Perish
The reduced levels of oxygen at altitude lead to something called hypoxia.
Interestingly, hypoxia tends to make you feel better, rather than worse. And when I say better, I mean even better than usual.
You become a bit tipsy, you hallucinate, you accept your situation, you even start to welcome it.
There is a BBC documentary called, ‘How to Kill a Human Being‘, claims the most humane way to kill someone is through hypoxia.
The scientists suggest it’s the only path to death that’s remotely close to being ‘pleasant’.
And that’s what you have to deal with as a mountaineer with AMS; a welcoming invitation to perish.
You’ve probably heard about mountaineers in the Himalaya walking aimlessly, stripping off their clothes, and lying around like it’s a day on the beach. Well, that’s hypoxia and AMS.
Hypoxia is experienced at altitude because the atmospheric pressure becomes lower as altitude increases. And with lower atmospheric pressure, we get less oxygen. Keep in mind, the percentage of oxygen in the air doesn’t decrease (air still contains about 21% oxygen), but the density of the air decreases.
On the summit of Everest (8,848m), the amount of oxygen in one breath is about a third of that at sea level. So in every breath, we receive two-thirds less oxygen, plus we’re exerting ourselves by climbing in heavy gear, up and down rugged terrain, and in the most inhospitable climatic conditions.
Can’t We Just Breathe Quicker?
Sure, that’s what ends up happening; we breathe quicker to take in more air. But, the problems don’t stop there. As we increase altitude, the amount of water in our lungs increases, which reduces the space for oxygen.
At just over 19,000m, your lungs would be full of water.
That means it wouldn’t even matter if you were breathing pure oxygen because there would be nowhere for it to go.
This happens for two completely separate reasons:
- As water enters our lungs, it becomes saturated by moisture in our bodies. The amount of water saturation increases at altitude.
- As our bodies notice the reduced oxygen, certain blood vessels in the lungs constrict to force blood into healthier parts. This constriction and pressure lead to water secreted into the lungs.
So, on top of having two-thirds less oxygen at the summit of Everest, we have less room in our lungs to hold inspired air and less surface area to absorb oxygen. Let’s look at the numbers:
- At sea level, the air in the lungs is about 6% water vapor
- This rises to just below 20% on Mt Everest’s summit
- So, you have about 14% less lung capacity to fill with oxygen
- Add that to having two-thirds less oxygen due to atmospheric pressure
- Now, we have about 25% of the oxygen that’s normally available at sea level
In technical-speak, this is measured by the partial pressure of oxygen inside and outside the lungs. Water has a constant partial pressure. So if you compare the same volume of moist and dry air, the moist air has a lower partial pressure and less available oxygen.
But Wait, There’s More!
Atmospheric pressure forces air into our mouths, through our alveoli, into our capillaries, and into our bloodstream. When atmospheric pressure drops, there’s less pressure to push oxygen into our blood.
Now, after finding we have only 25% of the oxygen available at sea level, we find there’s less pressure to force that oxygen into our blood.
The technical terms for this process is a gas exchange. It involves the switch between carbon dioxide and oxygen in our lungs across various membranes. This process becomes much less efficient at altitude because our bodies rely on atmospheric pressure to push gases through these membranes.
Luckily, our bodies increase the chemicals used to bind oxygen to deoxygenated hemoglobin. It gets even more technical, but for all of these responses, our bodies work overtime.
Mountaineering Stresses Our Bodies Beyond Belief
Mountaineering is more than just walking up hills. As mountain researcher, Lawrence Martin, suggests,
Survival [in mountaineering]Â is only possible because of… sustained hyperventilation.
To combat all of the negative effects of high altitude, we need to constantly breathe like it’s our last breath. Not only do we have to breathe quickly, but our heart rate must increase to pump blood (with much less oxygen) around the body to support the activity of climbing. Add to this the extreme cold, and mountaineers really do operate on the precipice of death.
Have you ever seen a mountaineering documentary and thought, “I wouldn’t have stopped. I would have kept going.” Well, it’s often not the climber’s conscious decision. Imagine sprinting until your heart rate is above 200 beats per minute. Now imagine standing at rest with 200 beats per minute. That’s mountaineering at high altitude.
The limit of a mountaineer is when his/her resting heart rate meets his/her maximal heart rate. When this happens, movement is virtually impossible.
So just to recap the issue of high altitude on the body:
- Problem #1:Â There’s less air at altitude, and hence less oxygen in each breath we take.
- Problem #2: There’s more water in our lungs, therefore less room for absorbable oxygen.
- Problem #3:Â Lower atmosphere pressure leads to less efficient gas exchange.
- Problem #4:Â The heart can only compensate until we hit the maximal heart rate.
Happy climbing đ